Travel Restrictions and the Omicron Variant
Amy Hurford, Maria M. Martignoni, Paul Tupper, Caroline Colijn, Sarah P. Otto, Christopher
McCabe and David J.D. Earn
2021-12-08
The Omicron variant (BA.1 and its sister lineage BA.2, formerly B.1.1.529.1 and B.1.1.529.2) was designated by the World Health Organization (WHO) as a variant of concern (VOC) on 26 November 2021 (World Health Organization 2021b) as it contains a large number of novel mutations, which may enhance its transmissibility, immune escape, partial resistance to vaccines, and severity profile (Torjesen 2021). Preliminary evidence also suggests an increased risk of reinfection (World Health Organization 2021b). Where monitored, Omicron has spread extremely quickly (e.g., South Africa, Botswana, and Ghana). In South Africa, Omicron was first detected on November 8, 2021, yet it constituted 73% of November genome sequencing data (National Institute for Communicable Diseases 2021). As of December 7th, Omicron has been detected in 57 countries worldwide (GISAID 2021).
Travel restrictions need to be implemented based on science (World Health Organization 2021c), and decisions that are not assessed through an evidence-informed approach will divide the international community, undermining global information sharing and solidarity, making the pandemic more harmful for all nations. Given these shortcomings, it is important to evaluate which specific actions provide the best balance between benefits and costs, and when the implementation of travel restrictions to reduce the spread of the Omicron variant is necessary and beneficial. Here we offer a summary of the considerations and a decision tree to help guide this assessment.
Travel restrictions can focus on either reducing the rate at which infected travellers arrive (the importation rate) or reducing the probability that imported cases generate community infections (Box 1). A reduction in the importation rate can be achieved by prohibiting entry of non-residents and/or flights arriving from specific countries, as long as the virus or VOC is predominantly in only those countries. Reducing the probability of initiating community spread is the main goal of restrictions such as arrival testing and self-isolation and quarantine requirements (World Health Organization 2021c). The associated efficacies and costs of different travel restrictions are described in Table 1.
Box 1
Modelling infection spread from travellers to members of the local community
Let the number of infected travellers with a vaccination status, \(i\), that arrive on a given day, \(t\), be \(n_i(t)\), where the time dependence, \(t\), is due to seasonal trends in travel volume and infection dynamics at the origin of arriving travellers. Each traveller has a probability, \(p_i(t)\), of infecting a member of the local community, where \(p_i(t)\) depends on the measures in place for travellers (i.e., see (Table 1)) with vaccination status i,
\[ p_i(t) = m_i\left(x_0(t) + z_1(t)x_1(t) + z_2(t)x_2(t)\right) \]
and also on vaccination levels in the local community, \(x_j(t)\), and vaccine effectiveness against infection, \(z_j(t)\) (where \(i,j=0\) unvaccinated, \(i,j=1\) is partially vaccinated, and \(i,j=2\) fully vaccinated). Here \(m_i\) is the probability that a traveller with the vaccination status \(i\) infects an unvaccinated community member given the post-arrival travel restrictions that are in place.
Each day, the number of infections spread from a traveller to a member of the community follows some distribution, for example, a binomial distribution, \(\text{BIN}(n_i(t), p_i(t))\) (Steyn et al. 2021). As such, on day \(t\), if nothing were to change in the future, the expected time until the first case is \(1/(n_i(t) p_i(t))\), and both reducing the importation rate, \(n_i(t)\), or reducing the probability that a traveler will initiate a community infection, \(p_i(t)\), will delay community spread within a jurisdiction.
More generally the modelling framework that has been developed to understand infection risk at gatherings might be applied to understand the spread of infection from travellers to members of a community. This may include estimating the event reproduction number (Tupper et al. 2020) for travellers that attend a community event, the probability that at least one community member is infected by an infected traveller (Champerdon, Fazil, and Ogden 2021), and by considering superspreading by assuming an overdispersed distribution such as the negative binomial (i.e., Endo et al. 2020).
Table 1
Possible measures for travelers to prevent the introduction of new variants. Assumptions: The virus is detectable by PCR test three days after infection with 100% accuracy (Crozier et al. 2021). Infectious individuals are detected with a rapid antigen test with 90% accuracy (Crozier et al. 2021). We assume screening for symptoms is in place. These measures are intended to detect asymptomatic or presymptomatic COVID cases. Different travel restrictions may apply to essential workers, the mobile workforce, residents, and arrivals by land versus air.
Measure | Efficacy | Cost |
---|---|---|
No action. | Zero. | Fastest community spread. | Negative PCR test required before travel | Moderate. Infections acquired from two days before the test to after the test will not be detected. Decreases both the travel volume and the number of travellers arriving infected (Public Health Agency of Canada 2021a). | Prevents travel among those who are detected. Cost of PCR test. |
PCR test required on arrival, quarantine if positive | Moderate. Infections acquired within the last two days of arrival will not be detected. | Cost of PCR test. Quarantine for positive cases. |
Rapid antigen test every day for five days after arrival. Verify (with repeated rapid test or PCR) and quarantine if positive. | Moderate to high. Rapid tests will be less sensitive than PCR, but is most sensitive for infectious individuals, preventing onward transmission when individuals isolate upon receiving positive test results. | Cost of rapid tests, risk of false positives. Quarantine for positive. |
Quarantine or self-isolation on arrival, PCR test after three days, leave quarantine or self-isolation on negative test. | High. Cases acquired abroad will be detectable with this delay. Efficacy at preventing onward infection depends on the efficacy of quarantine. Mandatory quarantine facilities may reduce travel volume if capacity is limited, while self-isolation efficacy depends on compliance. | Cost of PCR test. Quarantine for all individuals, with infection or not. |
Travel Ban | Complete (for the group to which the ban applies; essential travel will continue). | Prevents most travel, with infection or not. |
While travel bans may decrease the travel volume, such measures may not decrease the importation rate significantly if the countries targeted are not the ones with high prevalence (or high prevalence among potential travellers) (World Health Organization 2021a). It is challenging to know which countries are most affected, and the highest burden may not be in the locations where the virus is most detected. The situation is dynamic, and the list of countries known to be affected can change very rapidly, limiting the effectiveness of geographic travel bans. Furthermore, restrictions in the form of blanket travel bans have the disadvantage of affecting economies, limiting the flow of resources (including laboratory reagents and materials for essential research to understand the spread of Omicron and the threat it represents), affecting essential travel and reducing the sense of global solidarity. It may even disincentivize countries to report and share epidemiological data (World Health Organization 2021c). As such, there are strong reasons to implement travel restrictions in forms that allow entry to a region but reduce the probability of initiating spread from infected travellers to the local community.
Past SARS-CoV-2 data can also guide effective travel policies going forward. For example, introductions of VOCs to Canada have primarily come from the United States. From February 17-23, 2021 , the estimated highest risk of VOC importation was due to travellers from the USA infected with the Alpha variant (Public Health Agency of Canada 2021b), so excluding travellers from the United States from border measures is likely to miss a major source of introductions. Other data indicate that while only 20-30% of travellers to Canada arriving by air were exempt from 72 hours pre-arrival testing, these exemptions accounted for more than half the arriving infections (Public Health Agency of Canada 2021).
Deciding whether, and which, travel restrictions to apply may depend on characteristics of the new VOC (Figure 1) and of the jurisdiction (Box 2). Necessary conditions for travel restrictions to be warranted are: (1) the emergent variant presents concerning features or the jurisdiction is vulnerable due to low resources or capacity; (2) the risk of importing the variant from other jurisdictions warrants the restrictions; and (3) the VOC has not established or (4) can be eliminated because cases are so low (Baker, Wilson, and Anglemyer 2020).
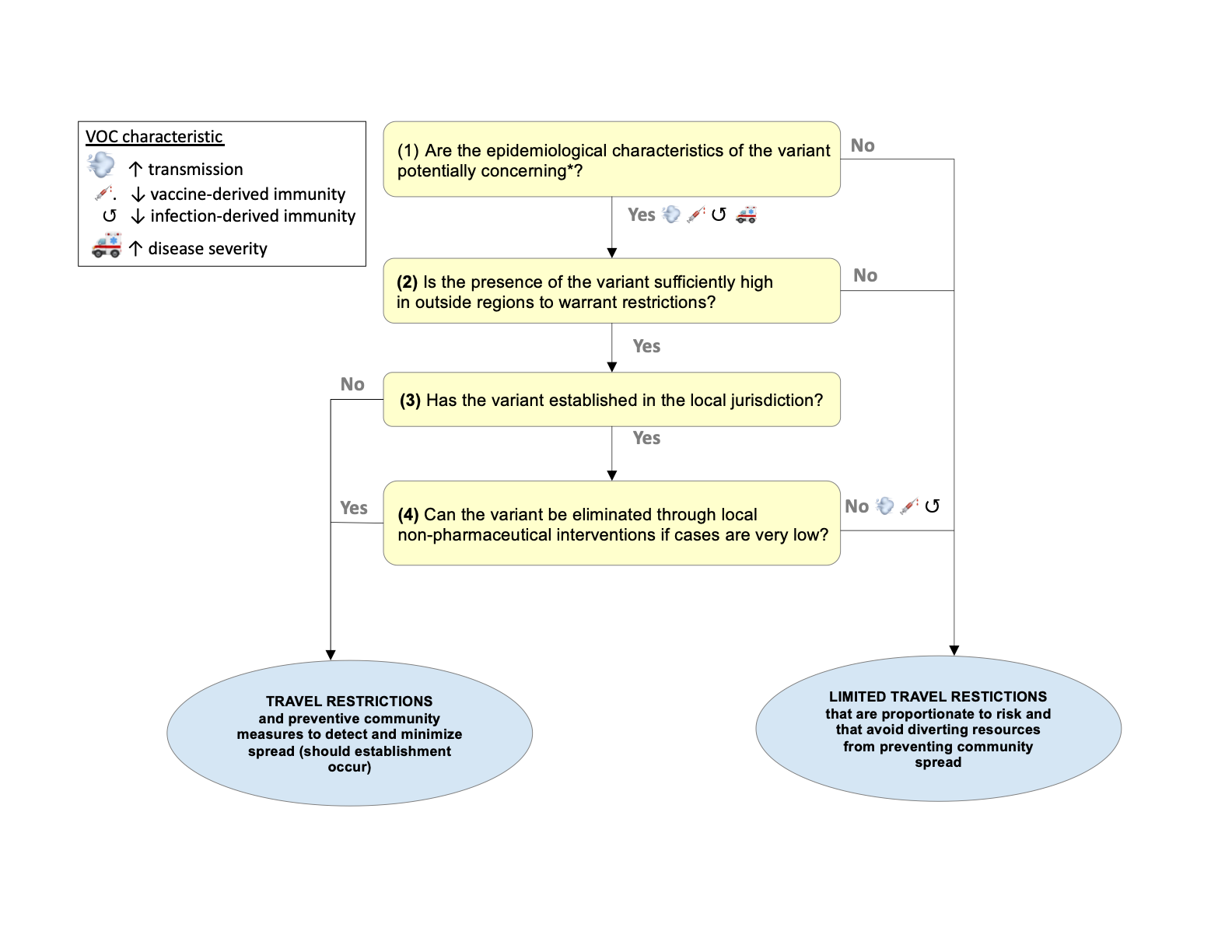
Figure 1
A decision tree to guide travel restrictions when new variants emerge. *Characteristics of the local jurisdiction, such as resource capacity, the prevalence of comorbidities, and immunity may affect whether the variant is considered potentially concerning.
Box 2
Travel restrictions and smaller jurisdictions
Smaller jurisdictions may have lower capacities, lower travel volumes, and geographical and social characteristics that reduce the transmission rate. All of these features favour the implementation of travel restrictions (Figure 1).
In small jurisdictions, hospital, contact tracing, and testing capacities may be low, such that capacity is overwhelmed shortly after exponential growth establishes. Travel restrictions may be implemented to ensure that the number of cases remains low and that contract tracing capacity is not exceeded (Tupper, Otto, and Colijn 2021).
When travel restrictions are in place, it may be possible to eliminate an outbreak before another importation establishes and leads to another community outbreak (Martignoni et al. in prep). In smaller jurisdictions, elimination may be possible due to a lower transmission rate as there are fewer offices and apartments accessed by elevators, less public transit, fewer large gatherings, and less anonymity, which increases contact tracing efficacy.
Variants A VOC that is more transmissible will threaten the contact tracing, testing, and hospital capacities of smaller jurisdictions, while a VOC that causes more severe disease will threaten the hospital capacity, and VOCs with both characteristics may warrant the implementation of travel restrictions. However, as for large jurisdictions, it may not be possible to eliminate a VOC that is more transmissible in a small jurisdiction, and should this occur, resources should not be diverted away from managing a community outbreak, to manage infections at the border (World Health Organization 2021a).
Initially, characteristics of the VOC may be unknown, such that it is considered ‘potentially serious’ until further investigations are completed. Travel restrictions can be warranted if they delay introduction and allow the development of additional evidence to support longer-term decision making or preparation. If the establishment of a new variant can be delayed for 2–3 weeks, we can expect more reliable estimates of the epidemic growth rate, for a broader range of populations, with different current outbreaks, as well as preliminary information from antibody neutralization studies (Kupferschmidt 2021) that will inform estimates of re-infectivity rates and vaccine effectiveness, all of which can support more targeted and appropriate policy responses, including whether travel restrictions should then be lifted, modified, or stay in place.
Once more is known about the VOC, characteristics that are concerning are increased transmissibility, erosion of vaccine-derived and infection-derived immunity, and increased disease severity. What is meant by ‘increased transmissibility’ is nuanced in populations with some immunity. By ‘transmissibility’ we mean spread between non-immune individuals, while increased transmission to vaccinated and recovered individuals, we refer to as the erosion of vaccine-derived and infection-derived immunity. For a VOC with any of these characteristics, its establishment is expected to spark large outbreaks, such that even if the proportion of the infected population that experiences severe disease remains the same, a greater number of severe disease outcomes may occur. If the proportion of the population that experiences severe disease increases, then the VOC may also be considered ‘concerning’. If the VOC is not found to have concerning characteristics, then travel restrictions are not needed.
If the variant is more transmissible, or if the VOC erodes infection-derived or vaccine-derived immunity, elimination in the local community may no longer be possible and travel restrictions might need to be lifted so that resources can be provided to the community (World Health Organization 2021a). The implementation of travel restrictions may be warranted in some jurisdictions but not others, as smaller jurisdictions may satisfy the conditions for the implementation of travel restrictions when larger jurisdictions do not (Box 2).
Conclusion
Travel restrictions can be warranted, especially when resource capacity is low or a new variant is threatening, and time is needed to gain information. Their implementation should be evidence-based, should enable a sense of global solidarity, promote international cooperation, and guarantee inclusion. If implemented, the need for travel restrictions should be continually reassessed. Once more is known regarding the epidemiological properties of the Omicron variant, the evidence should inform whether travel restrictions need to be continued or can be released.
References
Baker, Michael G., Nick Wilson, and Andrew Anglemyer. 2020. “Successful Elimination of Covid-19 Transmission in New Zealand.” New England Journal of Medicine 383 (8): e56. https://doi.org/10.1056/NEJMc2025203.
Champerdon, David, Amir Fazil, and Nicholas Ogden. 2021. “SARS-CoV-2 Transmission Risk at Gatherings: Mathematical Modelling Approaches, CCDR 47(4).” Can Commun Dis Rep 47 (4): 184–94.
Chinazzi, Matteo, Jessica T. Davis, Marco Ajelli, Corrado Gioannini, Maria Litvinova, Stefano Merler, Ana Pastore y Piontti, et al. 2020. “The Effect of Travel Restrictions on the Spread of the 2019 Novel Coronavirus (COVID-19) Outbreak.” Science 368 (6489): 395–400. https://doi.org/10.1126/science.aba9757.
Crozier, Alex, Selina Rajan, Iain Buchan, and Martin McKee. 2021. “Put to the Test: Use of Rapid Testing Technologies for Covid-19.” BMJ 372 (February): n208. https://doi.org/10.1136/bmj.n208.
Endo, Akira, Centre for the Mathematical Modelling of Infectious Diseases COVID-19 Working Group, Sam Abbott, Adam J. Kucharski, and Sebastian Funk. 2020. “Estimating the Overdispersion in COVID-19 Transmission Using Outbreak Sizes Outside China.” Wellcome Open Research 5 (April): 67. https://doi.org/10.12688/wellcomeopenres.15842.1.
GISAID. 2021. https://www.gisaid.org/.” December 7, 2021.
Kupferschmidt, Kai. 2021. “‘Patience Is Crucial’: Why We Won’t Know for Weeks How Dangerous Omicron Is.” 2021. https://www.science.org/content/article/patience-crucial-why-we-won-t-know-weeks-how-dangerous-omicron.
Martignoni, Maria M., Julien Arino, Troy Day, Proton Rahman, J.Concepcion Loredo-Osti, and Amy Hurford. In prep. “Optimal Public Health Responses to COVID-19 Differ in High and Low Cases Importation Regions.”
National Institute for Communicable Diseases. 2021. “SARS-CoV-2 GENOMIC SURVEILLANCE UPDATE.” NICD (blog). December 3, 2021. https://www.nicd.ac.za/diseases-a-z-index/disease-index-covid-19/sars-cov-2-genomic-surveillance-update/.
Public Health Agency of Canada. 2021a. “COVID-19: PHAC Modelling Group Report. April 22.”
———. 2021b. “COVID-19: PHAC Modelling Group Report. January 28.”
———. 2021. “COVID-19: PHAC Modelling Group Report. March 11.”
Steyn, Nicholas, Michael J. Plank, Alex James, Rachelle N. Binny, Shaun C. Hendy, and Audrey Lustig. 2021. “Managing the Risk of a COVID-19 Outbreak from Border Arrivals.” Journal of The Royal Society Interface 18 (177): 20210063. https://doi.org/10.1098/rsif.2021.0063.
Torjesen, Ingrid. 2021. “Covid-19: Omicron May Be More Transmissible than Other Variants and Partly Resistant to Existing Vaccines, Scientists Fear.” BMJ 375 (November): n2943. https://doi.org/10.1136/bmj.n2943.
Tupper, Paul, Himani Boury, Madi Yerlanov, and Caroline Colijn. 2020. “Event-Specific Interventions to Minimize COVID-19 Transmission.” Proceedings of the National Academy of Sciences 117 (50): 32038–45. https://doi.org/10.1073/pnas.2019324117.
Tupper, Paul, Sarah Otto, and Caroline Colijn. 2021. “Fundamental Limitations of Contact Tracing for COVID-19” 6 (1): 0016. https://www.sfu.ca/magpie/blog/fundamental-limitations-of-contact-tracing-for-covid-19.html.
World Health Organization. 2021a. “Technical Considerations for Implementing a Risk-Based Approach to International Travel in the Context of COVID-19: Interim Guidance : Annex to: Policy Considerations for Implementing a Risk-Based Approach to International Travel in the Context of COVID-19, 2 July 2021.” WHO/2019-nCoV/Risk-based_international_travel/2021.1. World Health Organization. https://apps.who.int/iris/handle/10665/342212.
———. 2021b. “Classification of Omicron (B.1.1.529): SARS-CoV-2 Variant of Concern.” November 26, 2021. https://www.who.int/news/item/26-11-2021-classification-of-omicron-(b.1.1.529)-sars-cov-2-variant-of-concern.
———. 2021c. “WHO Advice for International Traffic in Relation to the SARS-CoV-2 Omicron Variant (B.1.1.529).” November 30, 2021. https://www.who.int/news-room/articles-detail/who-advice-for-international-traffic-in-relation-to-the-sars-cov-2-omicron-variant.